- 1Center for the Study of Human Health, Emory University, Atlanta, GA, United States
- 2Department of Dermatology, Emory University School of Medicine, Atlanta, GA, United States
- 3Department of Biology, University of Pristina, Prishtinë, Kosovo
- 4Emory Antibiotic Resistance Center, Emory University, Atlanta, GA, United States
Hypericum perforatum L. (Hypericaceae), or St. John's Wort, is a well-known medicinal herb often associated with the treatment of anxiety and depression. Additionally, an oil macerate (Oleum Hyperici) of its flowering aerial parts is widely used in traditional medicine across the Balkans as a topical wound and ulcer salve. Other studies have shown that Oleum Hyperici reduces both wound size and healing time. Of its active constituents, the naphthodianthrone hypericin and phloroglucinol hyperforin are effective antibacterial compounds against various Gram-positive bacteria. However, hyperforin is unstable with light and heat, and thus should not be present in the light-aged oil macerate. Additionally, hypericin can cause phototoxic skin reactions if ingested or absorbed into the skin. Therefore, the established chemistry presents a paradox for this H. perforatum oil macerate: the hyperforin responsible for the antibacterial bioactivity should degrade in the sunlight as the traditional oil is prepared; alternately, if hypericin is present in established bioactive levels, then the oil macerate should cause photosensitivity, yet none is reported. In this research, various extracts of H. perforatum were compared to traditional oil macerates with regards to chemical composition and antibacterial activity (inhibition of growth, biofilm formation, and quorum sensing) vs. several strains of Staphylococcus aureus in order to better understand this traditional medicine. It was found that four Kosovar-crafted oil macerates were effective at inhibiting biofilm formation (MBIC50 active range of 0.004–0.016% v/v), exhibited moderate inhibition of quorum sensing (QSIC50active range of 0.064–0.512% v/v), and contained detectable amounts of hyperforin, but not hypericin. Overall, levels of hypericin were much higher in the organic extracts, and these also exhibited more potent growth inhibitory activity. In conclusion, these data confirm that oil macerates employed in traditional treatments of skin infection lack the compound credited with phototoxic reactions in H. perforatum use and exhibit anti-biofilm and modest quorum quenching effects, rather than growth inhibitory properties against S. aureus.
Introduction
Hypericum perforatum L. (Hypericaceae), or St. John's Wort, is a well-known medicinal herb regularly associated with the treatment of anxiety, historically determined by the ancient Greek physicians Pliny and Hippocrates (Blumenthal, 2002). The plant grows up to 1 m in height and features yellow flowers, rounded leaves, and oblong petals populated with a number of brown-black glandular dots, giving the plant its eponymous “perforated” appearance (WHO, 1999), Figure 1. It is native to Europe and Asia, while also having spread as an invasive species in North America and Oceania. Often used to treat depression and other mood disorders (Ng et al., 2017), dietary supplements featuring St. John's Wort reached an annual sale of $6 million in the United States in 2015 (Smith et al., 2016). H. perforatum is well-characterized chemically: many secondary metabolites have been identified, including naphthodianthrones (hypericin), phloroglucinols (hyperforin), flavonoid glycosides (hyperoside), biflavones, and anthocyanidins (Porzel et al., 2014). Currently, many compounds are now understood in mechanism and function; for example, antidepressant activity has been attributed to hyperforin, which acts as a reuptake inhibitor for neurotransmitters such as dopamine, norepinepherine, serotonin, and glutamate (Chatterjee et al., 1998) and is now used in the standardization of many Hypericum-based products sold on the commercial market (USP, 2015).
FIGURE 1
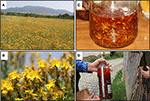
Figure 1. Traditional production of H. perforatum oil macerates for topical applications. (A) Plants are collected from the wild. (B) The flowering aerial parts are harvested. (C)The flowers are covered with oil (typically olive oil or sunflower oil) and exposed to the sun for 40 days. (D) The oil is ready for use once it has passed the sun exposure period and has taken on a blood red color.
Additionally, an oil macerate of H. perforatum flowers (Oleum Hyperici) is widely used as a traditional remedy across Bosnia and Herzegovina, Turkey, and Serbia for skin ulcers and burns (Kültür, 2007; Redžić, 2007; Šarić-Kundalić et al., 2010; Savikin et al., 2013), as well as in Kosovo (Mustafa et al., 2012a,b, 2015). The documentation of continued usages of the oil across the Balkan Peninsula stands in contrast to its less common use in the West. Historic literature indicates a past appreciation for the oil; The Eclectic Dispensatory of the United States of America quoted it as being “a fine red balsamic ointment for wounds, ulcers, swellings, tumors, etc.” in 1852 (King, 1852), and Potter's Cyclopaedia of Botanical Drugs and Preparationsdescribed it as “a healing application to wounds, sores, ulcers, and swellings” to Londoners in 1907 (Wren, 1907). Recent research showed that polysaccharides from H. perforatum have antimicrobial activity against Escherichia coli, Shigella dysenteriae, Salmonella typhi, Bacillus cereus, and Staphylococcus aureus when tested by a disk diffusion assay (Heydarian et al., 2017). Another study demonstrated H. perforatum extracts and partitions had antibacterial activity against several oral bacteria including Streptococcus mutans, S. sobrinus, Lactobacillus plantarum, and Enterococcus faecalis (Suntar et al., 2016).
Oleum Hyperici is prepared in the Balkan region by covering the flowering aerial parts of the plant in olive or sunflower oil in a transparent container (glass or plastic) and exposing to sunlight for at least 40 days until it turns an intense red color (Mattalia et al., 2013), Figure 1. Recent research has shown that Oleum Hyperici reduces both external wound size and healing time as an antibacterial (Suntar et al., 2010). The phloroglucinol hyperforin and naphthodianthrone hypericin (Figure 2) are reported to be responsible for its antibacterial activity (Saddiqe et al., 2010), effective as antibacterial compounds against various Gram-positive bacteria (Schempp et al., 1999), including Bacillus subtilis, E. faecalis, Staphylococcus epidermidis, and Micrococcus luteus (Marcetic et al., 2016), while ineffective against Gram-negative bacteria (Gibbons et al., 2002). Many compounds other than the naphthodianthrone and phloroglucinol derivatives have been identified in H. perforatum flowers; including the flavonol hyperoside, biflavonols such as amentoflavone and biapigenin, low levels of xanthone derivatives, common phenolic acids such as caddeic acid, chlorogenic acid, and ferulic acid, tannins and catechin derivatives are also present at significant concentrations (Dostalek and Stark, 2012; Matei et al., 2015).
FIGURE 2
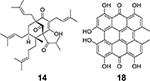
Figure 2. Structures of two bioactive compounds, hyperforin (14) and hypericin (18), examined in our chemical analysis of the H. perforatum extracts and traditional formulas.
Nevertheless, phloroglucinols are quite unstable with light and heat (Orth et al., 1999; Ang et al., 2002), and thus should not be present in an aged oil macerate of H. perforatum. Hyperforin, specifically, is susceptible to oxidation into furohyperforin, which is completely ineffective when treating Gram-positive and Gram-negative bacteria (Miraldi et al., 2006). Additionally, hypericin can cause phototoxic skin reactions if ingested or absorbed into the skin (Kamuhabwa et al., 1998), known as “hypericism”—a common pharmaceutical warning for fair-skinned individuals taking Hypericum supplements or evidenced by livestock that develop extreme photosensitivity after grazing on H. perforatum flowers (Genter, 2001)—as UV-activated hypericin has been demonstrated to kill human keratinocytes and melanocytes by means of necrosis and apoptosis (Davids et al., 2008). While hypericin is often cited as the main photosensitizing agent, pseudohypericin and hyperforin may also contribute to the phototoxicity of H. perforatumpreparations (Onoue et al., 2011). Although hypericin in a H. perforatum extract or in combination with other compounds found in H. perforatum, such as chlorgenic acid, can exhibit a lower phototoxicity than the hypericin alone (Schmitt et al., 2006).
Therefore, the established chemistry presents an interesting paradox to this traditional preparation of H. perforatum. The hyperforin thought to be responsible for much of the antibacterial bioactivity should degrade in the sunlight as the remedy is prepared, unless the oil increases the stability of certain terpenoids as it is known to do (Boskou, 1978) that, in turn, act as sacrificial antioxidants for hyperforin. Alternately, if hypericin is present in established bioactive levels and contributes to the oil's red colorization, then the traditionally prepared oil macerate should cause photosensitivity, yet none is reported. Here, we approach two central questions: (1) Do traditionally formulated Oleum Hyperici from the Balkans contain the phototoxic compound, hypericin; and (2) Does the anti-staphylococcal activity of various H. perforatum formulations differ? Based on a lack of skin sensitization reports following use of the oil (data from previous field studies in the Balkans) and its traditional use in treating skin infection, we hypothesize that Oleum Hyperici does not contain hypericin, but does exhibit antibacterial activity against the most common cause of skin infection, S. aureus. In this research, organic and aqueous extracts of H. perforatum as well as commercial dietary supplements (tablet and liquid tincture) were compared to four Kosovar oil macerates in both chemical composition and antibacterial activity against S. aureus in order to investigate the contradictory nature of this traditional medicine.
Experimental Methods
Collection and extraction of H. perforatum Samples
Plant material was collected following standard guidelines for collection of wild specimens (WHO, 2003), while H. perforatum olive oil, sunflower oil, unknown oil A, and unknown oil B macerates were procured from local Prizren, Kosovo markets and households in this region by CQ and AH. All procured oils were reported to have been created in the traditional method, with the flowering aerial parts covered in vegetable oil and exposed to sunlight for 40 days. Voucher specimens were deposited at the Emory University Herbarium (Accession Numbers: 20051 and 20091) and University of Prishtina Herbarium (BP-0002); digital copies of the specimens are accessible for viewing online via the SERNEC web portal (SERNEC, 2017). St. Johns Wort dietary supplements were purchased from a commercial vendor in Atlanta, Georgia, USA.
Shade-dried, aerial parts of H. perforatum were ground in a Thomas Scientific Wiley Mill (Swedesboro, NJ) through a 2 mm mesh. Dry powder (75 g) was transferred into an amber bottle and macerated in 750 mL of methanol (MeOH). The jug was wrapped in aluminum foil and left in the dark for 48 h, with agitation every 24 h. The MeOH extract was filtered through coarse and then fine filter paper. The marc was returned to the bottle and underwent a second 48 h maceration with 750 mL of MeOH and filtered as before. The filtrates were combined and evaporated using a rotary evaporator at <40°C. The dried extract was re-dissolved in DI water, shell frozen, and freeze-dried overnight on a Labconco FreeZone 2.5 lyophilizer (Kansas City, MO). All solvents were acquired from Fisher Chemical, Certified ACS grade (Pittsburg, PA). The dry extract was scraped and transferred to scintillation vials wrapped in aluminum foil, and then stored at −20°C. All of the above procedures were conducted under aluminum foil tents to reduce ambient light exposure. An aqueous extract of the same material was also obtained by boiling 40 g of ground plant material in 400 mL DI water. This decoction was then strained through cheese cloth, double filtered, concentrated, freeze dried, and collected similarly to the above organic extract.
The commercial St. John's Wort dietary supplement extract was indicated to contain 300 mg of St. John's Wort Extract (flower) per capsule standardized to 0.3% hypericin. Three of these capsules were emptied and pooled, with 1.1 g of the contained powder from three capsules dissolved in 4.5 mL of MeOH in an Eppendorf tube. The solution was sonicated under aluminum foil for 30 min and centrifuged at 3,000 rpm for 15 min. The supernatant was transferred to a round-bottom flask and concentrated by removal of MeOH with a rotary evaporator. A 10 mL aliquot of the commercial liquid St. John's Wort flower buds and tops tincture (65–75% USP alcohol, indicating a 500 mg mL−1 herb equivalency) was concentrated with a rotary evaporator. Both commercial extracts were concentrated, stored and tested under reduced light. In total, eight samples were prepared for this experiment: a MeOH extract of the aerial parts (MAP), a MeOH extract of a tablet supplement (TS), a concentration of an EtOH liquid supplement (LS), a decoction of aerial parts (HAP), an olive oil macerate (OOO), a sunflower oil macerate (SOO), a macerate of unknown oil A (UOA), and another macerate of unknown oil B (UOB), Table 1. Extracts were prepared for bacterial assays by dissolving in DMSO at 10 mg mL−1. Oil macerates and an olive oil control were prepared by first mixing with Tween20 (4:1), and then further diluting in media relevant to each bioassay (1:4), for a final 16% v/v oil emulsion. Tea tree essential oil was prepared at 10% v/v oil in an emulsion mixture (0.5% v/v Tween80 in DMSO) for use as a positive control.
TABLE 1
Characterization by HPLC and LC-FTMS
High performance liquid chromatography (HPLC) analysis was conducted on an Agilent Technologies ZORBAX Eclipse XDB-C18 250 × 4.6 mm, 5 μm column (Santa Clara, CA) with a compatible guard column at a column temperature of 25°C, monitored at 588 nm for extracts and 254 nm for oil macerates, adapting a method for a smaller column size by Liu (Liu et al., 2005). Runs were performed on an Agilent 1260 Infinity system using OpenLab CDS ChemStation. Reagents were HPLC grade and purchased from Fisher Scientific, with the exception of the Type 1 DI water, which was obtained from an EMD Millipore MILLI-Q water system (Billerica, MA). Mobile phases consisted of: (A) 20 mM ammonium acetate in water and ACN (9:1) and (B) ACN. The flow rate was 1 mL/min using samples at a 100 mg mL−1concentration. Samples were prepared using HPLC and MS-grade MeOH, while oil macerates were analyzed after being dissolved in ethyl acetate (4:1). The injection volume was 5 μL, with gradient elution beginning at 50% B, increasing linearly from 2 to 22 min to 100% B, holding at this concentration for 9 min, before returning to initial conditions for 9 min.
The above parameters were also used for liquid chromatography Fourier transform mass spectrometry (LC-FTMS) experiments. Samples were run on a Shimadzu SIL-ACHT (Tokyo, Japan) and Dionex 3600SD HPLC pump (Sunnyvale, CA), with data acquired in MS mode scanning from an m/z of 150–1,500 on a Thermo Scientific LTQ-FT Ultra MS in both negative and positive ESI modes and processed with Thermo Scientific XCalibur 2.2 SP 1.48 software (San Jose, CA). The capillary temperature was 275.0°C, sheath gas of 60, source voltage of 5.0 kV and current 100.00 μA, and the capillary voltage −19.0 or +32.0 V, respectively. Peaks featuring putative compounds of the oil macerates were identified throughout the entire chromatogram and searched across the Dictionary of Natural Products (CRC Press) and Scifinder (Chemical Abstracts Service). High resolution masses of compounds were determined from the LC-FTMS data and searches conducted throughout the databases for all compounds identified in H. perforatum within a similar mass range. Additionally, an authentic standard of hyperforin DCHA (AdipoGen Corp., Sandiego CA) with ≥97% purity was analyzed by the previously described LC-FTMS method to aid in identification of this compound in the various H. perforatum preparations.
UV-Vis Analysis
An Agilent Cary 50 UV-Vis Spectrometer was used to obtain UV-Vis spectra from 190 to 1,100 nm of the Oleum Hyperici samples and an olive oil control sample (Badia Extra Virgin Olive Oil). The data was collected and spectra analyzed using WinUV and Microsoft Excel.
Antibacterial Evaluation
Growth Inhibition Assay
S. aureus cultures (described in Table 2) were grown in Tryptic Soy Broth (TSB), with cation-adjusted Mueller Hinton broth (CAMHB) used for minimum inhibitory concentration (MIC) evaluation following standard Clinical and Laboratory Standards Institute (CLSI) methods (CLSI, 2013). To present a detailed view of the growth inhibitory activity across all samples tested, the MIC50 and MIC90, defined as the concentration required for at least 50 or 90% inhibition of growth, were both reported. The MIC90 is equivalent to the “MIC,” defined as the concentration required for no visible growth in the well. All extracts and Oleum Hyperici samples prepared in this study were examined for MIC values against S. aureus strains representing the four accessory gene regulator (agr) alleles (agrI: AH1677, agrII: AH430, agrIII: AH747, agrIV: AH1872) to observe potential trends in the inhibition of quorum sensing activity, MRSA strain LAC (AH1263), as well as a biofilm test strain (UAMS-1). All concentrations were tested in triplicate and repeated twice on different days. Controls included the vehicles (DMSO and olive oil emulsion), tea tree oil as an antibacterial oil control (Thursday Plantation, Australia), and antibiotics Vancomycin and Ampicillin (MP Biomedicals, Santa Ana, CA). Overnight cultures were standardized by optical density (OD) to 5 × 105 CFU mL−1, and this was confirmed by plate counts of colonies. MIC50 and MIC90 values were assigned as described (Quave et al., 2015); this was determined by reading plates at an OD600 nm in a Cytation 3 multimode plate reader (BioTek, Winooski, VT) after 18 h incubation. In addition, MIC values of oil macerates were determined on an oil macerate volume/final well volume% basis (compared to μg mL−1 reporting for organic and aqueous extracts).
TABLE 2
Quorum Sensing Inhibition Assay
Quorum sensing activity was investigated as described (Quave and Horswill, 2014; Quave et al., 2015), using previously described (Kirchdoerfer et al., 2011) S. aureus agr P3-YFP reporter strains (AH1677, AH430, AH1747, AH1872; Table 2). Briefly, overnight cultures grown in TSB supplemented with chloramphenicol (Cam) were diluted in fresh media with Cam to yield a final well starting inoculum of 5 × 105 CFU mL−1. Black sided microtiter plates (Costar 3603) were incubated at 37°C with shaking (1,200 rpm) in a Stuart SI505 incubator (Bibby Scientific, Burlington, NJ) with a humidified chamber. Readings at OD600 nm and fluorescence (top reading, 493 excitation, 535 nm emission, gain 60) were taken after 18 h incubation. Controls included vehicles (DMSO and olive oil emulsion) and 224C-F2, a previously reported quorum sensing inhibitor (Quave et al., 2015). Inhibition of quorum sensing activity for oil macerates was determined on a volume/volume% basis (compared to μg mL−1 reporting for organic extracts). All extracts were tested at sub-inhibitory concentrations for growth, as determined in MIC assays.
To determine if any observed quorum sensing inhibition was influenced by potential growth inhibitory effects of the test agents, growth and fluorescence was monitored in parallel at multiple time points over a 20 h period. Furthermore, colony counts were taken at 18 h post incubation by serial diluting and plating aliquots of treatment and control groups in triplicate onto TSA using the drop-plate method (10 μL drops). Plates were incubated for 12 h, after which dilution factors with 3–30 colonies present per drop were counted to determine final CFU mL−1for each group.
Biofilm Inhibition Assay
Anti-biofilm activity was investigated using a human plasma protein-coated assay as previously described (Beenken et al., 2010; Quave et al., 2012) with S. aureus strains UAMS-1 and its isogenic sarA mutant (UAMS-929) as a biofilm deficient phenotypic control (Beenken et al., 2003). Briefly, following inoculation and addition of media (containing extract or vehicle alone) with a starting inoculum of 5 × 105 CFU mL−1, 96-well plates (Falcon 35-1772) were incubated for 22 h at 37°C, washed with phosphate-buffered saline (PBS), fixed with ethanol, stained with crystal violet and rinsed in tap water. The stain was then eluted into the ethanol and was transferred to a new plate prior to quantification of the eluate at an OD595 nm. The MBIC50 and MBIC90 are defined here as the minimum concentration of test agent required to inhibit 50 or 90% of biofilm formation, respectively. Controls included vehicles (DMSO and olive oil emulsion), tea tree oil as an oil comparison, and 220D-F2, a previously reported biofilm inhibitor (Quave et al., 2012).
Statistical Analysis
All tests were performed in triplicate and repeated on at least two different occasions. Heteroscedastic Student's t-tests were performed in Microsoft Excel and significance is denoted for all tests at *P < 0.05, †P < 0.01, and ‡P < 0.001.
Results
Growth Inhibition
HAP (aqueous extract of flowering aerial parts; see Table 1) and MAP (methanol extract of flowering aerial parts) exhibited the strongest growth inhibitory activity across all S. aureusstrains examined, with MIC90 values of 128 and 8–32 μg mL−1, respectively (Figure 3). The Oleum Hyperici samples (OOO, SOO, UOA, UOB; see Table 1) did not exhibit growth inhibitory action at the concentration range examined (maximum of 0.512% v/v), with the exception of OOO, which exhibited an MIC50 of 0.512% v/v for one strain (AH1872). The commercial supplements (LS—liquid supplement and TS—tablet supplement) likewise exhibited only limited growth inhibitory activity; LS exhibited a MIC50 in the range of 64–256 μg mL−1 against three of the strains, and a MIC90 of 256 μg mL−1 against one. Dose-response data is presented in Figure 3and summary of MICs in Table 3. These findings concerning the lack of strong antibacterial activity by the commercial supplements (TS and LS) and Oleum Hyperici samples were also confirmed by multiple OD readings over a 20 h period (Figure 4) and colony counts (Figure 5).
FIGURE 3
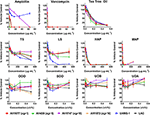
Figure 3. Growth inhibitory activity of H. perforatum extracts against strains of S. aureus. Activity was detected by measure of optical density of wells (OD600nm) and is reported as percent of the vehicle control. Positive controls included antibiotics (ampicillin and vancomycin) and tea tree oil.
TABLE 3
FIGURE 4
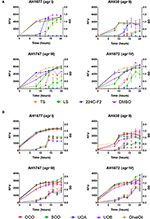
Figure 4. Impact of H. perforatum commercial supplements and Oleum Hyperici samples on growth and agr activity over 20 h as determined by optical density and fluorescence measures of four S. aureus agr reporters. Solid lines in the graphs denote OD; broken lines denote RFU. (A) Organic extracts and controls. (B) Oil macerates and controls. Test concentrations correspond with results on quorum sensing inhibition reported in Table 3. Briefly, TS and LS were tested at 32 μg mL−1, DMSO (vehicle control) at eight concentrations from 0.02 to 0.64% v/v, and 224C-F2 (positive control) at eight concentrations from 0.5 to 64 μg mL−1, with results from the highest concentrations for the vehicle and positive control reported here. For oil macerate tests, OOO was tested at 0.512% v/v for agr I and III, 0.064% for agr II, and 0.256% for agrIV; SOO was tested at 0.512% for agr I, III, and IV, 0.064% for agr II; UOA was tested at 0.512% for agr I, III, and IV, 0.0128% for agr II; UOB was tested at 0.512% for agr I, III, and IV, 0.0128% for agr II. Lastly, Olive Oil was tested at 8 concentrations from 0.004 to 0.512% and the highest concentration is reported here.
FIGURE 5
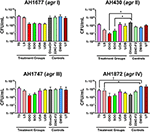
Figure 5. Impact of H. perforatum commercial supplements and Oleum Hyperici samples on S. aureus growth, as determined by colony counts. To further investigate whether the decreased level of quorum sensing activity in extracts and oil macerates was due to any potential growth inhibitory effects, the number of colony forming units (CFU) per mL of broth was determined at 18 h. Test concentrations correspond to those reported in Figure 4. Significant differences between the vehicle control and treatment groups are denoted as *P < 0.05, †P < 0.01, and ‡P < 0.001.
Quorum Sensing Inhibition
Modest inhibition of quorum sensing (QS) was observed in three (OOO, SOO, UOA) of the oil macerate samples against the agrII reporter (AH430), with QSIC50 values of 0.064–0.128% v/v, Table 3. All four oil samples inhibited QS in the agrIV isolate (QSIC50 0.256–0.512% v/v); SOO also inhibited QS in agrI (AH1677) at an IC50 of 0.512% v/v. Two of the organic extracts (TS and LS) inhibited quorum sensing (QSIC50 of 32 μg mL−1 against AH430), but this activity did not exhibit a dose-dependent improvement in activity with an increase in test concentration, Figure 6. Time-dependent examination of OD and relative fluorescence units (RFU) activity over a 20 h period revealed that the observed quorum sensing inhibitory activity was not an artifact of growth inhibitory effects of the samples. Colony counts at 18 h of incubation also confirmed this, with the exception of a minor—but statistically significant—difference in the number of colonies between the olive oil control and SOO and OOO samples for the agrII reporter strain and also between the DMSO vehicle control and LS and TS in the agrIV reporter strain (Figure 5). None of these exhibited a lower number of colonies in comparison to the vehicle controls in more than one strain.
FIGURE 6
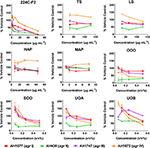
Figure 6. Impact of H. perforatum extracts on S. aureus quorum sensing, as detected by agr reporters. Activity was detected by measure of fluorescence and is reported as percent of the vehicle control. The botanical extract “224C-F2” (a known quorum quencher) was used as the positive control (Quave et al., 2015).
Impact on Biofilm Formation
All of the H. perforatum extracts (oils and organic extracts) exhibited some degree of inhibition of biofilm formation, Table 3 and Figure 7. Among the organic extracts, MAP was the most potent (MBIC50 of 8 μg mL−1), however, this extract also exhibited growth inhibitory effects (MIC50 of 8 μg mL−1). In all of the organic extracts, the growth inhibitory effects of the extracts were responsible for the diminished level of biofilm formed.
FIGURE 7
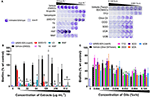
Figure 7. Impact of H. perforatum extracts on S. aureus biofilm formation, as detected by static microtiter plate crystal violet assay. USA 200 isolate UAMS-1 and its isogenic sarA mutant (UAMS-929) were used in the biofilm assay. (A) Images of crystal violet stained biofilm in 96-well plates. The optical density (OD595 nm) of the biofilm eluent is reported as percent of the wild type control (UAMS-1) for the (B) organic and (C) oil extracts. Significance is denoted as *P < 0.05, P < 0.01, and ‡P < 0.001.
With regards to the traditional oil macerates, biofilm inhibition was observed in all four oils (OOO, SOO, UOA, UOB) with MBIC50 values ranging from 0.004 to 0.016% v/v. This inhibition was observed in the absence of any growth inhibitory activity (max test concentration of 0.512% v/v).
Chemical Analysis
Percent yields for extracts are reported in Table 1. Analysis of the extracts by HPLC and LC-FTMS revealed the presence of hypericin in the organic and aqueous extracts (MAP, TS, LS, HAP), but not in the oil macerate samples (OOO, SOO, UOA, UOB), Tables 4, 5. Exact mass data of a total of 46 compounds was collected (Table 5).
TABLE 4
TABLE 5
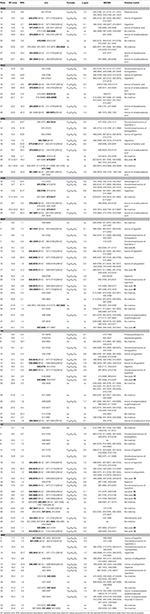
Table 5. Mass spectrometry (MS) and MS/MS analysis of the peak data for oil macerates as reported in Figure 8.
LC-FTMS analysis of the oil samples revealed the presence of 12 distinct compounds, only four of which were found in all four samples, Figure 8; this included the putative compounds: constitutional isomer of furohyperforin (12), hyperforin (14), and isomers of octadecadienoic acid (21 and 40), Table 5. Compounds 21 and 40 had the highest relative abundance of all components of the oil macerate samples. Peaks 13, 14, 20, 23, and 44 all had m/z of 535.38, by comparison of the retention times and mass spectra with that of an authentic standard it was determined that 14 was hyperforin. Peaks 13 and 14 also have similar MS2 fragments. Since hyperforin has been identified as having 3 tautomers, 1,3 diketone and two enols, the authors have assigned 13 as the enol form. This tautomerization was shown to occur on HPLC columns at a pH of 2.5, very similar to the pH of the 0.1% formic acid mobile phases used in these HPLC methods (Fourneron and Naït-Si, 2006; Lee et al., 2006). Peaks 20, 23, and 44 all have base peaks with m/z 535.38; however, the MS2 is not consistent with hyperforin and these peaks have been assigned as constitutional isomers of hyperforin.
FIGURE 8
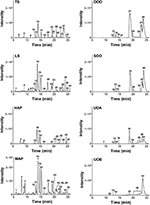
Figure 8. Characterization of H. perforatum extracts by LC-FTMS. Chromatograms of organic and oil extractions of H. perforatum are reported; peak numbers correspond to Table 5.
Analysis of the organic and aqueous samples revealed the presence of six compounds common in all four samples: 12, 14, isomer of adhyperforin (17), hypericin (18), hyperforin (23), and 43; compounds 12 and 14 were the only ones also present in the oil samples. Peak 20 (an isomer of hyperforin) was present in all of the organic extracts (TS, LS, MAP), but absent in the aqueous extract (HAP).
Discussion
Chemistry
Through LC-FTMS analysis of different H. perforatum formulations, we have demonstrated that the traditional oil macerate (Oleum Hyperici), used in topical applications for skin and soft tissue infections throughout the Balkans, contains the bioactive compound hyperforin, but not the photosensitizing compound, hypericin. In contrast, the MeOH, aqueous, and commercial supplement extracts all featured both hypericin and hyperforin. The composition difference between the two classes of sample in this study is most likely due to the methods of their extractions, with photo-extraction in an oil solution yielding very different compounds than a low-light organic or aqueous extract. This supports field observations of a lack of reported photosensitive reactions among users of the oil for topical skin care.
However, in contrast to our findings concerning the chemistry of Oleum Hyperici, Miraldi et al. (2006) presented a different case, in that hyperforin, adhyperforin, hyperevolutin A and B, hypericin and pseudohypericin are entirely absent from preparations of this oil macerate. These results were found in oil macerates produced by 15 days of sun exposure—as opposed to 40, as described in ethnobotanical research (Mustafa et al., 2015). Nevertheless, the presence of hyperforin—a photolabile, oxidation prone phloroglucinol derivative—in a formulation which relies on extended periods of sun exposure presents some additional questions. Mainly, how is it possible that this compound remains stable in this traditional preparation? One potential explanation based on the work of Boskou (1978) might be that the abundant terpenoids in the oil itself could be stabilized in light and heat, and in turn, act as sacrificial reducing agents for the ROS preventing the eventual oxidation of hyperforin into furohyperforin. While this change can be evaluated by an increase in the oil's viscosity due to an increase ratio of palmitic to linoleic acid, further experimentation and research is required to evaluate this change in the oil. The degradation of hyperforin follows first order kentics yielding a tautomeric mixture of 2-methyl-3-hydroxy-4-(1-oxo-2-methyl-1-propyl)-1,5-dioxo-6-(3methyl-1-but-2-enyl)-2-cyclohexeneand 2-methyl-1,3,5-trioxo-4-(1-hydroxy-2-methyl-1-propylene)-6-(3-methyl- 1-but-2-enyl)-cyclohexane via three Norrish type II reactions (D'Auria et al., 2008). The oil or other compounds in the oil macerate may be absorbing some of the light necessary for the Norrish reactions to occur, thus preventing the hyperforin from decomposing. An analysis of a purchased olive oil sample by UV-Vis showed a strong absorption band at 299–302 nm (data not shown) and the spectrum below this wavelength was very noisy indicating that the oil itself has a strong and complex absorbance in this range. Published UV-Vis spectra of hyperforin show a λ of 298–310 nm depending on the solvent (Vuong et al., 2011; Ng et al., 2017). Based on this analysis, the olive oil itself is absorbing UV light in the range that is necessary to degrade the hyperforin and thus protecting the compound in the oil maceration.
Other factors that can contribute the variations in the oil macerates include the natural variation in the H. perforatum plant material used to make the preparations in the different studies. These variations are known to influence both the chemical composition and bioactivity of Hypericumproducts (Marrelli et al., 2014). Variations in secondary metabolite concentrations could be the result of the botanical source material being grown in differing climates or having been exposed to differing stressors, such as herbivory, infection, or drought. Due to such potential variation, any commercial preparation of H. perforatum should be standardized to established marker compounds using accepted analytical methods, those published by AOAC [AOAC Stakeholder Panel on Strategic Foods Analytical Methods (SPSFAM), 2013].
Bioactivity
We demonstrated with our bioactivity findings that while the traditional oil macerates (Oleum Hyperici) do not exhibit strong anti-staphylococcal growth inhibitory activity as is noted in the organic and aqueous preparations of the flowering aerial parts, they do exhibit biofilm inhibitory properties at sub-inhibitory concentrations for growth and exhibit modest quorum quenching effects against three of the four accessory gene regulator (agr) alleles. The quorum sensing inhibitory activity of the ethyl acetate extract of a related species (H. connatum) has been reported against Pseudomonas aeruginosa (Fratianni et al., 2013). In previous work, we assessed the capacity of H. perforatum and many other Mediterranean species to inhibit production of delta-hemolysin, a transcriptional product controlled by the agr system, however the ethanolic extract of H. perforatum stems exhibited only mild inhibitory activity (36% inhibition of delta-toxin production; Quave et al., 2011). Thus, to the best of our knowledge, this is the first report of a Hypericum species extract or formulation inhibiting quorum sensing at a level >50% against S. aureus.
The growth inhibitory activity of the extracts containing hypericin (MAP, TS, LS, HAP) was unsurprising as hypericin is reported to be the major growth inhibitory antibacterial agent for this species (Saddiqe et al., 2010; Yow et al., 2012). In addition to numerous studies on the growth inhibitory properties of H. perforatum extracts, a few have also examined their anti-biofilm potential. Extracts of the adventitious roots have demonstrated anti-biofilm activity against the fungus Malassezia furfur (Simonetti et al., 2016). With regards to anti-biofilm activity in bacteria, hyperforin and its hydrogenated analog have been shown to inhibit planktonic and biofilm cultures of S. aureus and E. faecalis (Schiavone et al., 2013). A suite of Hypericum spp. secondary metabolites were found to exhibit anti-biofilm activities against S. aureus and S. epidermidis, one of which—a phloroglucinol from H. punctatum—had an MBIC (no detectable biofilm formation) of just 1.95 μg mL−1 (Sarkisian et al., 2012).
In our analyses, we found that the organic, aqueous, and oil extracts all exhibited some level of biofilm inhibition against S. aureus. However, it was difficult to gain an accurate assessment of the inhibitory activity for biofilm formation in the organic and aqueous extracts (HAP and MAP) as this was confounded by their strong growth inhibitory activity. On the other hand, the oil macerates (OOO, SOO, UOA, UOB), which lacked the strongly antibacterial hypericin, did demonstrate statistically significant inhibition of biofilm formation in the absence of growth inhibition. Early work with H. perforatum attributed antibacterial activity to hyperforin which was present in all the oil macerates (Gurevich et al., 1971). While hypericin has stronger antibacterial activity, hyperforin has reported MICs and minimum bactericidal concentrations (MBC) as low as 1 mg mL−1 by agar-diffusion assays for some preparations against S. aureus(Schempp et al., 1999). Based on studies concerning the anti-biofilm effects of hyperforin (Schiavone et al., 2013), its MIC, and our documentation of appreciable levels of hyperforin in the traditional oil macerate, could explain—at least in part—the activity observed. Importantly, while other studies have described the anti-biofilm activity of isolated compounds and other Hypericum extracts, this is the first report of the biofilm inhibitory activity of the traditional Oleum Hyperici formulation. This data concerning the antibacterial (anti-biofilm and quorum quenching) activity combined with the absence of the harmful photosensitizing agent hypericin, provide compelling evidence of valid efficacious and safe use of this traditional remedy.
Conclusions
Different formulations of H. perforatum flowering aerial parts are used in traditional medicine, Western herbalism, and in dietary supplements. In this study, we aimed to investigate the safety and antibacterial efficacy of an oil macerate formulation (Oleum Hyperici), which is one of the most common topical therapies for skin and soft tissue infections used in the Balkans as compared to other organic and aqueous extractions, and commercial supplement preparations of the plant. We determined that the traditional preparation of Oleum Hyperici, which involves 40 days of sun exposure in oil, results in a product that lacks the phototoxic naphthodianthrone compound hypericin, responsible for skin sensitization reactions and hypericism, supporting our hypothesis. Our hypothesis concerning the antibacterial activity of Oleum Hyperici was refuted in some assays, and supported in others. Specifically, oil macerates did not inhibit bacterial growth overall, but did significantly inhibit biofilm formation and quorum sensing, which is responsible for the recalcitrant nature of S. aureus infections and the regulation of a suite of harmful staphylococcal toxins, respectively. This suggests that the traditional Oleum Hyperici formulation may have more utility in regulation of staphylococcal virulence and pathogenesis rather than classic antibiotic activity.
In conclusion, we have demonstrated that this topical folk-medical therapy for skin and soft tissue infections (including ulcers and wounds) could represent a safe and efficacious therapy for further development. Perhaps the most important consequence of this study is the chemical and biological validation of a traditional medicine, which could continue to play an important role in human medicine in the future. From a union of ethnobotany and biochemical analyses, these findings corroborate the biological mechanism of efficacy of this treatment.
Author Contributions
AH, BM, and CQ: Collected the material; JL and CQ: Conceived and designed the experiments; AB, AK, CQ, JL, and KN: Performed the experiments and analyzed the data; AK, JL, and CQ: Prepared the draft; All authors proofread the final draft and approved the final manuscript.
Funding
This work was supported by a grant from the National Institutes of Health, National Center for Complementary and Integrative Health (R01 AT007052, PI: CQ) and the US Embassy in Kosovo (S-KV-420-14GR-096). The content is solely the responsibility of the authors and does not necessarily reflect the officinal view of NCCIH, NIH or the US Department of State. The funding agencies had no role in the study design, data collection and analysis, decision to publish, or preparation of the manuscript.
Conflict of Interest Statement
The authors declare that the research was conducted in the absence of any commercial or financial relationships that could be construed as a potential conflict of interest.
Acknowledgments
The authors would like to thank Dr. Fred Strobel of the Department of Chemistry at Emory University for assistance with the mass spectrometry experiments. We thank Dr. Mark Smeltzer for providing strain UAMS-1 and Dr. Alexander Horswill for the agr reporter strains. We also thank Dr. Cora MacBeth and Dr. Savita Sharma for the use of the UV-Vis Spectrometer and assistance in running these samples.
References
Ang, C. Y. W., Cui, Y., Chang, H. C., Luo, W., Heinze, T. M., Lin, L. J., et al. (2002). Determination of St. John's Wort components in dietary supplements and functional foods by liquid chromatography. J. AOAC Int. 85, 1360–1369. Available online at: http://aoac.publisher.ingentaconnect.com/contentone/aoac/jaoac/2002/00000085/00000006/art00020
Fratianni, F., Nazzaro, F., Marandino, A., Fusco Mdel, R., Coppola, R., De Feo, V., et al. (2013). Biochemical composition, antimicrobial activities, and anti–quorum-sensing activities of ethanol and ethyl acetate extracts from Hypericum connatumLam. (Guttiferae). J. Med. Food 16, 454–459. doi: 10.1089/jmf.2012.0197
Quave, C. L., Lyles, J. T., Kavanaugh, J. S., Nelson, K., Parlet, C. P., Crosby, H. A., et al. (2015). Castanea sativa (European Chestnut) leaf extracts rich in ursene and oleanene derivatives block Staphylococcus aureus virulence and pathogenesis without detectable resistance. PLoS ONE 10:e0136486. doi: 10.1371/journal.pone.0136486
Redžić, S. S. (2007). The ecological aspect of ethnobotany and ethnopharmacology of population in Bosnia and Herzegovina. Coll. Antropol. 31, 869–890. Available online at: http://hrcak.srce.hr/26902
SERNEC (2017). Southeast Regional Network of Expertise and Collections [Online]. Available line at: http://sernecportal.org/portal/ (Accessed 3 April 2017).
Simonetti, G., Tocci, N., Valletta, A., Brasili, E., D'Auria, F. D., Idoux, A., et al. (2016). In vitro antifungal activity of extracts obtained from Hypericum perforatum adventitious roots cultured in a mist bioreactor against planktonic cells and biofilm of Malassezia furfur. Nat. Prod. Res. 30, 544–550. doi: 10.1080/14786419.2015.1028059
Smith, T., Kawa, K., Eckl, V., and Johnson, J. (2016). Sales of Herbal Dietary Supplements in US Increased 7.5% in 2015 Consumers spent $6.92 billion on herbal supplements in 2015, marking the 12th consecutive year of growth. HerbalGram111, 67–73. Available online at: http://cms.herbalgram.org/herbalgram/issue111/hg111-mktrpt.html
Keywords: Hypericum perforatum, Staphylococcus aureus, traditional medicine, phototoxicity
Citation: Lyles JT, Kim A, Nelson K, Bullard-Roberts AL, Hajdari A, Mustafa B and Quave CL (2017) The Chemical and Antibacterial Evaluation of St. John's Wort Oil Macerates Used in Kosovar Traditional Medicine. Front. Microbiol. 8:1639. doi: 10.3389/fmicb.2017.01639
Received: 01 March 2017; Accepted: 14 August 2017;
Published: 08 September 2017.
Published: 08 September 2017.
Edited by:
Tzi Bun Ng, The Chinese University of Hong Kong, Hong Kong
Reviewed by:
Chien-Yi Chang, Guangzhou Women and Children Medical Center, ChinaBen W. Greatrex, University of New England, Australia
Atte Von Wright, University of Eastern Finland, Finland
Catherine M. T. Sherwin, University of Utah, United States
Sunil D. Saroj, Symbiosis International University, India
Copyright © 2017 Lyles, Kim, Nelson, Bullard-Roberts, Hajdari, Mustafa and Quave. This is an open-access article distributed under the terms of the Creative Commons Attribution License (CC BY). The use, distribution or reproduction in other forums is permitted, provided the original author(s) or licensor are credited and that the original publication in this journal is cited, in accordance with accepted academic practice. No use, distribution or reproduction is permitted which does not comply with these terms.
*Correspondence: Cassandra L. Quave, cquave@emory.edu
†These authors have contributed equally to this work.